About Author: Mr.Mahendra G. Pawar* (B.Pharmacy) Ms.Komal R. Nikam (B.Pharmacy), Mr.Rakesh D.Amrutkar (M.Pharmacy)
Reference ID: PHARMATUTOR-ART-1059
Abstract
Biotechnologists in recent years have come up with a new concept.This new concept is about edible vaccine. The difference here lies, that crops like “golden rice” provided extra nutrition that naturally didn’t occur in it. But edible vaccines are GM crops that would provide extra added “immunity” from certain diseases.
Edible vaccines are composed of antigenic proteins and do not contain pathogenic genes (because obviously they use attenuated strains). Thus, they have no way of establishing infection and safety is assured.Oral administration is possible , production is highly efficient and can be easily scaled up.For example, hepatitis-B antigen required to vaccinate whole of China annually, could be grown on a 40-acre plot and all babies in the world each year on just 200 acres of land,Cheaper (single dose of hepatitis-B vaccine would cost approximately 23 paise), grown locally using standard methods and do not require immense capital investment of pharmaceutical manufacturing facilities, exhibit good genetic stability. Do not require special storage condition. Since syringes and needles are not used chances of infection are also less. Fear of contamination with animal viruses - like the mad cow disease, which is a threat in vaccines manufactured from cultured mammalian cells - is eliminated, because plant viruses do not infect humans.
Introduction
Edible Vaccines have many potential advantages:(21)
Edible plants are very effective as a delivery vehicle for inducing oral immunization.
Adjuvant for immune response is not necessary.
Excellent , feasibility of oral administration compared to injection.
Easy for separation and purification of vaccines from plant materials.
Effective prevention of pathogenic contamination from animal cells.
Convenience and safety in storing and transporting vaccines.
Effective maintenance of vaccine activity by controlling the temperature in plant cultivation.
Easy for mass production system by breeding compared to an animal system.
Possible production of vaccines with low costs.
Reduced need for medical personnel and sterile injection conditions.
Economical to mass produce and transport.
Reduced dependence on foreign supply.
Storage near the site of use.
Heat stable, eliminating the need for refrigeration.
Antigen protection through bioencapsulation.
Subunit vaccine (not attenuated pathogens) means improved safety.
Disadvantages:(21)
Dosage of vaccines would be variable.
Not convenient for infants.
Limitations:
Producing stable and reliable amounts of vaccines in plants is complicated by the fact that tomatoes and bananas don’t come in standard sizes! There may also be side-effects due to the interaction between the vaccine and the vehicle. People could ingest too much of the vaccine, which could be toxic, or too little, which could lead to disease outbreaks among populations believed to be immune.
Side Effects of Edible Vaccine:(62)
Development of edible vaccines is a possible high-volume, low-cost delivery system for third-world countries to fight against fatal maladies like AIDS, hepatitis and diarrhea. Research by the National Institute of Allergy and Infectious Diseases (NIAID) and the University of Maryland School of Medicine showed no significant side effects in a small study using genetically-engineered potatoes to make toxin of the Escherichia coli, a diarrhea-causing bacterium.
No Serious Side Effects:
Volunteers reported no serious adverse reactions to genetically-altered potatoes used to deliver edible vaccine toxin, according to the National Institutes of Health. The NIH said 10 to 11 volunteers who ate the raw potato bites developed four times the antibodies against the E. coli bacteria without obvious side effects.
Reduced Anaphylactic Risk:
Reduced risk of anaphylactic side effects from edible vaccine over injection systems is one benefit reported by the Bio-Medicine.org. They report the edible vaccine carries only part of the allergen compared to injection methods that reduce anaphylactic risk.
Possible Disease Spread:
Potential risk of spreading the disease by edible vaccine delivery is a concern of Mindfully.org. Potential contamination of the oral delivery system is a possible danger.
Delayed Reactions:
Long-term reactions to edible vaccines are yet to be determined. Possible delayed reactions not yet discovered are dangers the World Health Organization would like further study on before edible vaccines are put into production.
Objective:
The objective of present review article is to provide detailed information on a new dosage form of vaccines i.e. edible vaccines produced from transgenic plants. This review includes the study of edible vaccines, their advantages, and their future prospectus along with some examples.
Review:(50)
Edible vaccines hold great promise as a cost-effective, easy-to-administer, easy-to-store, fail-safe and socioculturally readily acceptable vaccine delivery system, especially for the poor developing countries. It involves introduction of selected desired genes into plants and then inducing these altered plants to manufacture the encoded proteins. Introduced as a concept about a decade ago, it has become a reality today. A variety of delivery systems have been developed. Initially thought to be useful only for preventing infectious diseases, it has also found application in prevention of autoimmune diseases, birth control, cancer therapy, etc. Edible vaccines are currently being developed for a number of human and animal diseases. There is growing acceptance of transgenic crops in both industrial and developing countries. Resistance to genetically modified foods may affect the future of edible vaccines. They have passed the major hurdles in the path of an emerging vaccine technology. Various technical obstacles, regulatory and non-scientific challenges, though all seem surmountable, need to be overcome. This review attempts to discuss the current status and future of this new preventive modality.
Vaccines have been revolutionary for the prevention of infectious diseases. Despite worldwide immunization of children against the six devastating diseases, 20% of infants are still left un-immunized; responsible for approximately two million unnecessary deaths every year, especially in the remote and impoverished parts of the globe. This is because of the constraints on vaccine production, distribution and delivery. One hundred percent coverage is desirable, because un-immunized populations in remote areas can spread infections and epidemics in the immunized "safe" areas, which have comparatively low herd immunity. For some infectious diseases, immunizations either do not exist or they are unreliable or very expensive. Immunization through DNA vaccines is an alternative but is an expensive approach, with disappointing immune response hence the search is on for cost-effective, easy-to-administer, easy-to-store, fail-safe and socioculturally readily acceptable vaccines and their delivery systems. As Hippocrates said, "Let thy food be thy medicine," scientists suggest that plants and plant viruses can be genetically engineered to produce vaccines against diseases such as dental caries; and life-threatening infections like diarrhea, AIDS, etc. This is the concept of edible vaccines. The following discussion will address issues relating to their commercial development, especially their usefulness in preventing infectious diseases in developing countries.
Mechanism of Action:(50)
The antigens in transgenic plants are delivered through bio-encapsulation, i.e. the tough outer wall of plant cells, which protects them from gastric secretions and finally break up in the intestines. The antigens are released, taken up by M cells in the intestinal lining that overlie peyer's patches and gut-associated lymphoid tissue (GALT), passed on to macrophages, other antigen-presenting cells; and local lymphocyte populations, generating serum IgG, IgE responses, local IgA response and memory cells, which would promptly neutralize the attack by the real infectious agent.
Preparation of Edible Vaccines:(50)
Fig.1: Preparation of Edible Vaccines
Introduction of foreign DNA into plant's genome can either be done by bombarding embryonic suspension cell cultures using gene-gun or more commonly through Agrobacterium tumefaciens, a naturally occurring soil bacterium, which has the ability to get into plants through some kind of wound (scratch, etc.). It possesses a circular "Ti plasmid" (tumor inducing), which enables it to infect plant cells, integrate into their genome and produce a hollow tumor (crown gall tumor), where it can live. This ability can be exploited to insert foreign DNA into plant genome. But prior to this, the plasmid needs to be disarmed by deleting the genes for auxin and cytokinin synthesis, so that it does not produce tumor. Genes for antibiotic resistance are used to select out the transformed cells and whole plants, which contain the foreign gene; and for expressing the desired product, which can then be regenerated from them.
The DNA integrates randomly into plant genome, resulting in a different antigen expression level for each independent line, so that 50-100 plants are transformed together at a time, from which one can choose the plant expressing the highest levels of antigen and least number of adverse effects. Production of transgenic plants is species dependent and takes 3-9 months. Reducing this time to 6-8 weeks is currently under investigation. Some antigens, like viral capsid proteins, have to self-assemble into VLPs (virus-like particles). VLPs mimic the virus without carrying DNA or RNA and therefore are not infectious. Each single antigen expressed in plants must be tested for its proper assembly and can be verified by animal studies, Western blot; and quantified by enzyme-linked immunosorbentassay (ELISA).
Approaches to mucosal vaccine formulation include (i) gene fusion technology, creating non-toxic derivatives of mucosal adjuvants; (ii) genetically inactivating antigens by deleting an essential gene; (iii) co-expression of antigen and a cytokine, which modulates and controls mucosal immune response; and (iv) genetic material itself, which allows DNA/RNA uptake and its endogenous expression in the host cell. Various mucosal delivery systems include biodegradable micro- and nanoparticles, liposomes, live bacterial/viral vectors and mucosal adjuvants. "Prime-boost" strategy combines different routes of administration and vaccine types, especially where multiple antigens or doses are required. For example, a single parenteral dose of MV-H DNA (measles virus haemagglutinin) followed by multiple oral MV-H boosters could induce greater quantities of MV-neutralizing antibodies than with either vaccine alone.
Vaccines on a plate?(49)
Generally antibodies are sensitive to temperature, and so the material would have to be eaten raw. Dr Julian Marr (one of the leading scientists in this field) produces his vaccine against oral bacteria in a potato- one that must not be cooked! However, this crunchy vegetable is not the only option; there are potential vaccine candidates in bananas, maize, tomato and tobacco plants.
Another problem is ensuring the vaccine survives the digestive processes of the gut. One possible method would be to use attenuated (i.e. rendered harmless) strains of gut bacteria such as Salmonella. However, as with any attenuated organism, this could carry some risk. An alternative method would be to encapsulate the antibodies so that they are protected from the digestive chemicals in the gut. One option would be to naturally encapsulate the antibody within seeds- which are slowly digested.
One aspect that biotechnology companies would also need to consider would be how effective these vaccines actually are. Currently, most experimental vaccines do not provide adequate protection with just one dose, and biotech companies are looking at ways to boost this immune response. The immune response could be increased by adding an adjuvant (i.e. another chemical which boost the immune response).
What are edible vaccines? How are they manufactured?(19)
Fig.2: Edible vaccine
In this case, edible vaccines are antigenic proteins that are genetically engineered into a consumable crop. The idea is that the crop food product contains the protein which is derived from some disease causing pathogen. People eat the crop, the food is digested, and some of the protein makes its way into the blood stream. Get enough of this protein into the blood stream and it causes an immune response. This immune response would now nuetralize the pathogen should the person ever encounter it in the future.The nice thing about edible vaccines is that it's a very cheap way to produce vaccines, and it's also a very convenient way to store, ship and distribute vaccines. Not to mention, kids generally like eating more than getting shots. The problem is that ingestion is not always a reliable means of adminstering a vaccine. It's hard to get enough protein where it needs to be to get a good immune response.
Production:(47)
While plant system may have the capability of producing any vaccine in large amounts and in a less expensive manner, purification of the product may require the use of existing or even more cumbersome procedures. Attention therefore has been paid to mainly those antigens that stimulate mucosal immune system to produce secretory IgA (S-IgA) at mucosal surfaces, such as gut and respiratory epithelia. In general, a mucosal response is achieved more effectively by oral instead of parenteral delivery of the antigen. Thus, an antigen produced in the edible part of a plant can serve as a vaccine against several infectious agents which invade epithelial membranes. These include bacteria and viruses transmitted via contaminated food or water, and resulting in diseases like diarrhoea and whooping cough.
The first report of the production of edible vaccine (a surface protein from Streptococcus) in tobacco, at 0.02% of total leaf protein level, appeared in 1990 in the form of a patent application published under the International Patent Cooperation Treaty. Subsequently, a number of attempts were made to express various antigens in plants. Since acute watery diarrhoea is caused by enteroxigenic Escherichia coli and
Fig.3: Strategies for expression of antigens in plants.(47)
Vibrio cholerae that colonize the small intestine and produce one or more enterotoxin, an attempt was made towards the production of edible vaccine by expressing heat-labile enterotoxin (LT-B) in toabacco and potato.
Table 1: Antigen produced in transgenic plants(21)
The enterotoxin (LT) from E. coli is a multimeric protein, quite similar to cholera toxin (CT) structurally, functionally and antigenically. LT has one A subunit (27 kDa) and a pentamer of B subunits (11.6 kDa). Binding of the non-toxic LT-B pentamer to GM1 gangliosides, present on epithelial cell surfaces, allows entry of the toxin LT-A subunits into the cells. LT-B and CT-B are both potent oral immunogens. An oral vaccine composed of the cholera toxin-B subunit (CT-B) with killed V. cholerae cells has been reported to give significant level of protection against cholera26. But the cost of production of CT-B by conventional methods is too high to allow distribution of this vaccine. The recombinant LT-B (rLT-B) produced in tobacco and potato showed partial pentamerization after the engineering of subunit gene in a way that allowed retention of the protein in microsomal vesicles. On testing immunogenicity of rLT-B by feeding potato tubers to mice, both humoral and mucosal immune responses were reported to be stimulated. This vaccine has gone through pre-clinical trials in humans. The antigenic protein retained its immunogenecity after purification from the transgenic potato expressing it. Fourteen healthy individuals, who ate 50–100 g raw potatoes, were screened for gut-derived antibody secreting cells (ASC), which were detectable 7–10 days after immunization. Presence of both anti-LT IgA-secreting cells and anti-LT IgG-secreting cells was detected in the peripheral blood.
Cholera toxin, which is very similar to E. coli LT, has also been expressed in plants. Hein et al. generated tobacco plants expressing CT-A or CT-B subunits of the toxin. CT-A produced in plant was not cleaved into A1 and A2 subunits, which happens in epithelial cells. Plants expressing CT-B showed the presence of a protein that migrated to the same position in denaturing gel as the CT-B derived from V. cholerae, and was recognized by mouse anti-CT-B antibody. Cholera toxin-B subunit, when expressed in potato, was processed in a natural way: the pentameric form (the naturally occurring form) being the abundant form. Antigenically it was found to be similar to the bacterial protein. Even after boiling transgenic potato tubers till they became soft, approximately 50% of the CT-B was present in the pentameric GM1 ganglioside-binding form.
Similarly, a rabies virus coat glycoprotein gene has been expressed in tomato plants. The protein that was expressed had molecular mass of 62 kDa compared to 66 kDa observed from virus grown in BHK cells. Since the orally administered protein elicited protective immunity in animals, it was expected that continued efforts would lead to development of an edible oral vaccine against rabies which could be used as a preventive strategy. While the results with antigenic properties of the components produced in plants are encouraging, their value as a vaccine can be improved by providing other adjuvants which either enhance the immunogenic potential or reduce the degradation of the active ingredient by the gut microflora.
The Hepatitis B surface antigen (HBsAG) has been reported to accumulate to 0.01% of soluble protein level in transgenic tobacco. The antigens, delivered in a macromolecular form, are known to survive the gut atmosphere and perform better. Interestingly, the recombinant HBsAG was recovered in virus-like particles of 22 nm diameter (similar to yeast-derived HBsAG-based vaccine) which is known to be a prerequisite for better immunogenicity. A crude extract from plants was used for parenteral immunization in mice. The immune response included all IgG subclasses as well as IgM against hepatitis B. Carrillo et al. expressed structural protein, VP1, of foot-and-mouth disease virus in Arabidopsis. The mouse that was immunized intra-peritoneally with a leaf extract elicited immune response to synthetic peptides carrying various epitopes of VP1, or to complete VP1. Furthermore, all the mice immunized with the leaf extract were protected against challenge with virulent foot-and-mouth disease virus.
One of the alternative strategies of producing a plant-based vaccine is to infect the plants with recombinant viruses carrying the desired antigen that is fused to viral coat protein. The infected plants have been reported to produce the desired fusion protein in large amounts in a short time. The technique involved either placing the gene downstream a subgenomic promoter, or fusing the gene with capsid protein that coats the virus. The latter strategy is perhaps the strategy of choice since fusion proteins in particulate form are highly immunogenic. It should, however, be kept in view that recombinant viruses need to be highly purified for parenteral administration or partially purified for oral administration. Modelska et al. have shown that immunization of mice intraperitoneally or orally by gastric incubation or by feeding of plants infected with the recombinant alfalfa mosaic virus (AIMV) carrying rabies peptide CPDrg 24 mounted local as well as systemic immune response. Oral administration could stimulate both serum IgG as well as IgA synthesis. After immunization, 40% of the mice were protected against the challenge with a lethal dose of the virus.
Table 2: Transient production of antigens in plants after infection with plant viruses expressing a recombinant gene.(21)
Likewise, a 13-amino-acid epitope of zona pellucida, ZP3, protein and another epitope from malarial sporozoites have been expressed as fusion proteins with TMV capsid protein with the idea of developing anti-fertility and anti-malarial vaccines28. The antigenicity of the products has been found to be positive. The same is true for epitopes derived from human immunodeficiency virus which were expressed as alfalfa mosaic virus coat protein fusion products30. Recently, scientists at Axis Genetics, Cambridge, have shown that injecting mink with extracts of plants infected with a cowpea mosaic virus, that expresses a mink enteritis antigen gene, protects the animal against subsequent virus challenge31. While much remains to be done, indications are that plant-based vaccines can compete with vaccines produced by other approaches, particularly keeping in view the low cost and ease of production/distribution.
How to make an edible vaccine:
In four giant steps:
Pick a plant, like the tomato or potato, that can be easily engineered to express foreign proteins.
Engineer it to contain carefully selected DNA fragments (which let the plant produce the antigens) from the target germ.
Give the plant a signal so it will make the protein in its tissues.
Protein |
Plant |
Hepatitis B surface antigen Rabies virus glycoprotein Norwalk virus capsid protein E.coliheat-labile enterotoxin B subunit Cholera toxin B subunit Mouse glutamate decarboxylase VP1 protein of foot and mouth disease virus Insulin Glycoprotein swine-transmissible gastroenteritis cornavirus
|
Tobacco Tomato Tobacco Potato Potato, tobacco Potato Arabidopsis Potato Arabidopsis |
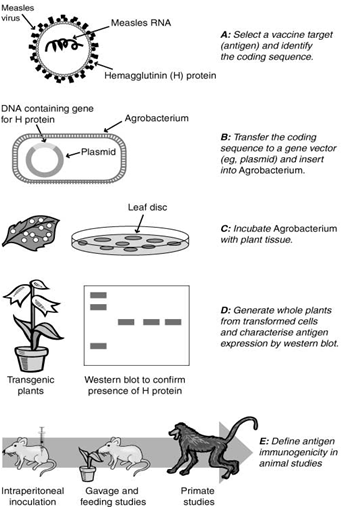
Fig 4:Strategy for the development of plant edible vaccine(61).
Vaccines and antibodies play a key role in healthcare. However, the cost of production and maintaining a chain for vaccine distribution has so far hampered realizing their full potential. Expression of antigens as vaccines, and of antibodies against antigens of pathogens in transgenic plants is a convenient and inexpensive source for these immunotherapeutic molecules. Various antigens and antibodies have already been expressed successfully in plants and have been shown to retain their native functional forms. Edible plant vaccine against diarrhoea, expressed in potato, and antibody against dental caries, expressed in tobacco, is already in pre-clinical human trials. Attempts are being made to express many proteins of immunotherapeutic use at high levels in plants and to use them as bio-reactors of the modern era.
Most of the drugs used by man, until very recently, were being derived from plants, which subsequently led to pharmaceutical companies starting chemical synthesis of the medicinal compounds. Recent progress in the area of transgenic plants has, however, once again attracted attention of the scientists, and plants are being looked upon as potential bio-reactors or bio-factories for the production of immunotherapeutic molecules. Transgenic material, in the form of seed or fruit, can be easily stored and transported from one place to another without fear of its degradation or damage. Furthermore, a large amount of bio-mass can be easily produced by cultivation in fields with relatively few inputs. In addition, transgenic plants capable of producing several different products can be created at any given time by crossing plants producing different products.
TARGET SPECIES FOR VACCINES |
PLANT USED FOR EXPRESSION |
ROUTE OF ADMINISTRATION |
Enterotoxigenic E.coli |
TOBACCO |
Oral |
Enterotoxigenic E.coli |
POTATO |
Oral |
Enterotoxigenic E.coli |
MAIZE |
Oral |
Vibrio cholerae |
POTATO |
Oral |
Hepatitis-B virus |
POTATO |
Oral |
Hepatitis-B virus |
LUPIN |
Oral |
Hepatitis-B virus |
LETTUCE |
Oral |
Norwalkvirus |
TOBACCO |
Oral |
Norwalkvirus |
POTATO |
Oral |
Rabies virus |
TOMATO |
-- |
Human cytomegalovirus |
TOBACCO |
-- |
Plant / Fruit |
Advantage |
Disadvantage |
|
Tobacco |
Good model for evaluating recombinant proteins. Low cost preserving system. Easy purification of antibodies stored in the seeds, at an location. Large harvests, number of times/year |
Produces toxic compounds.
|
|
Potato
|
Dominated clinical trials Easily manipulated/transformed Easily propagated from its “eyes” Stored for long periods without refrigeration |
Needs cooking which can denature antigen and decrease immunogenicity.
|
|
Banana
|
Do not need cooking Proteins not destroyed even if cooked Inexpensive Grown widely in developing countries
|
Trees take 2-3 years to mature Transformed trees take about 12 months to bear fruit Spoils rapidly after ripening Contains very little protein, so unlikely to produce large amounts of recombinant proteins |
|
Tomato
|
Grow quickly Cultivated broadly High content of vitamin A may boost immune response Overcome the spoilage problem by freeze-drying technology Heat-stable, antigen-containing powders***, made into capsules Different batches blended to give uniform doses of antigen |
Spoils readily
|
|
Rice
|
Commonly used in baby food because of low allergenic potential High expression of proteins/ antigens Easy storage/transportation Expressed protein is heat-stable |
Grows slowly Requires specialized glasshouse conditions
|
|
Lettuce Soybean
|
Fast-growing Direct consumption Large harvests, number of times/year
|
Spoils readily
|
Edible vaccine using tomato:(20)
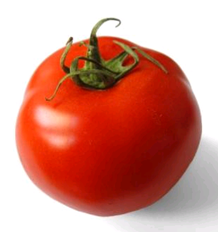
Fig.5: Tomato edible vaccine
Scientists took the process one step further by finding out whether or not the gene could be passed on to future generations of plants.
This testing process consisted of taking the seeds of the transgenic tomatoes and couching them. This was followed by cultivating a batch of second generation tomatoes, which were also transgenic and were found to contain the anti-gene protein.
• A possible edible vaccine against HIV/AIDS and hepatitis B.
• Cuts down the likelihood of the passing of infections.
• Inexpensive.
• Don't need special facilities for storage or transportation.
• They taste good.
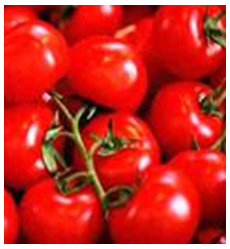
Fig.6: Tomato edible vaccine for HIV
Tomatoes serve as an ideal candidate for this HIV antigen because unlike other transgenic plants that carry the protein, tomatoes are edible and immune to any thermal process, which help retain its healing capabilities. Even more importantly, tomatoes were found to grow at a high rate of success in Russia, compared to bananas, another vaccine producing fruit already used.
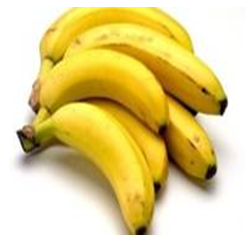
Fig.7: Banana vaccine
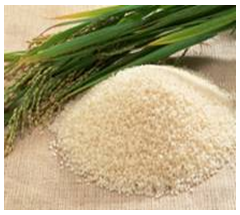
Fig.8: Edible vaccine using genetically modified rice
The genetic material from the microbe responsible for producing cholera toxin into a rice plant, whose genome has recently been sequenced. The plants produced the toxin and when the rice grains were fed to mice they provoked immunity from the diarrhea-causing bacterium. "We are considering rice as a new vaccine production and storage system, and natural vaccine delivery vehicle"The vaccine expressed in rice, or rice-based vaccine, will become a new form of vaccine production and delivery to [the] digestive tract for the initiation of antigen-specific mucosal and systemic immune responses."
Rice offers several advantages over traditional vaccines: it does not require needles, purification or refrigeration. In fact, the rice proved just as potent after 18 months of storage at room temperature and the vaccine did not dissolve when exposed to stomach acids, the researchers report in Proceedings of the National Academy of Sciences USA. But the immune response itself will require periodic updating. "Oral boosting should be necessary for the induction of antigen-specific immune response,"
Because rice grains contain varying amounts of the vaccine—roughly 30 micrograms per seed—a pill of some kind would need to be created to make sure people get the proper dose. The rice plants would also have to be grown under carefully controlled conditions to ensure appropriate vaccine production. "We do not have any plan to deliver the vaccine as a form of steamed rice, "A powder form of rice-based vaccine will be given in a tablet or capsule form."
The researchers point out that because rice plants do not scatter their pollen as widely as some of the other crops genetically modified to produce vaccines—corn, wheat, tomatoes—and are widely grown (unlike vaccine-producing bananas and potatoes), they pose less risk of contaminating normal crops and have broader utility. Such rice-based vaccines need not stop at cholera,. The same technique could be used to create rice grains bearing protection against the flu, botulism or anthrax, among other diseases. Someday, the adage may be: A bowl of rice a day, keeps the needle away.
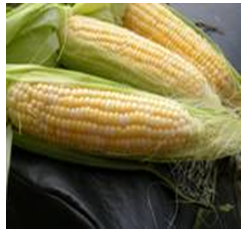
Fig.9: Edible vaccine using GM maize
GM maize plants that produce the protein known as HbsAg, which elicits an immune response against the hepatitis B virus and could be used as a vaccine.
To increase the amount of the protein produced by the plants. They have not yet tested the effectiveness of the edible vaccine in animals and humans, but expect that tests will start early next year.
Reporting the results at an international conference on genetic engineering, effective vaccine against the disease is vital, as many people are excluded from immunisation programmes because of the expense of the vaccines.
Edible vaccine for developing countries that would not require refrigerators for storage, or skilled medical personnel and needles to deliver the vaccine.
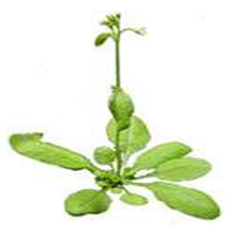
Fig.10: Edible vaccine used in HIV infection
The research group at Örebro Life Science Center has successfully transformed Arabidopsis thaliana and has gone on to produce several transformed plant lines, which express the protein corresponding to the inserted gene. We have also shown that the gene is inherited by the offspring. Hopefully, the protein expressed by these plants will elicit the immune system in the mucosal tissues during consumption. We are now carrying out trials with mice eating these transformed plants in order to establish whether they develop any immunological response.
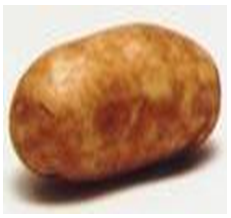
Fig.11: Potato vaccine
Researchers demonstrated that the edible vaccine is safe and stimulates antibodies, or germ-fighting proteins in the volunteers tested. We are excited about this novel approach to developing vaccines because of its potential to protect individuals around the world, especially in regions where injected vaccines are less practical an edible vaccine could be easy to produce, safe, affordable and effective.
To be immunized, people would need to eat a raw vegetable that contains the gene for the vaccine protein. It's exciting to think of the future for this type of vaccine delivery system and the varieties of organisms we may one day be able to fight.
The Norwalk Virus and closely related members of the same virus family account for more than 90 percent of non-bacterial gastroenteritis (severe abdominal pain and diarrhea) in developing countries, the rapid and severe spread of the Norwalk Virus is a leading cause of infant mortality.
A gene that encoded the Norwalk Virus coat into a potato plant's DNA. The potato vaccine was then tested at the Center for Vaccine Development in a double-blind study of 24 adult volunteers. Results show that 19 of the 20 volunteers (95 percent) who were given the potato containing the vaccine developed antibodies that fight the Norwalk Virus.
Edible plant vaccines will continue to be tested in volunteers for the next few years and could be available to protect people in 5-10 years. A potato vaccine against E. coli, a common cause of serious diarrhea in children and adults around the world. The study showed that eating the potato was an effective way to develop immunity to the E. coli toxin.
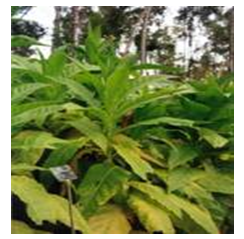
Fig.12: Edible vaccine using tobacco
Currently, more than 150 different types of Human Papillomaviruses are known. The most commonly found HPVs in cervical carcinomas are HPV 16 and 18. The cancer causing factors are the virus proteins E6 and E7, which are known as onco-proteins. Onco-proteins represent a promising target for the development of a therapeutic vaccine against HPV-associated tumors. This has been confirmed by an experiment showing that mice immunized with crude plant extracts containing the onco-protein E7 are partially protected against HPV-induced cancers.
Some E7-based HPV vaccines are currently being explored and the first promising results have been disclosed, but they still need further improvement to ensure protection. One method to produce high amounts of E7 protein in a relatively short time is by genetically engineering plants or plant viruses. “An advantage of the plant-derived systems is that they generally lack human pathogens, oncogenic DNA sequences and endotoxins “This minimizes health risks and lowers the production costs.”
Plants can be genetically modified by either stable or transient transformation. With stable transformation, the introduced DNA integrates in the genome of the plant, whereas with transient transformation, the introduced gene sequences do not integrate in the genome of the plant.
Ideal tools for transient expression are viruses, whose genomes contain, as an additionary gene, the sequences of interest. When the plant is infected with the virus, the virus spreads and replicates and the genes introduced in its genome are expressed in high amounts. The fact that viruses are usually not transmitted by pollen – which ensures genetic contamination of wild growing plants is prevented - is a further advantage of viral systems.
The HPV16 E7 protein in the cytoplasm of tobacco (Nicotiana benthamiana) plants. To do so, they used the potato virus X (PVX). PVX is a safe tool for genetic engineering, because it does not infect animals, but does effectively transfer genetic material to a variety of plant species.
Mice immunized sub-cutaneously with crude extracts of transgenic tobacco plants showed strong immune responses and about 40 percent of the animals were protected from HPV-induced tumors. The researchers hypothesized that the low percentage of responders could be due to the low amount of E7 antigen in the plant extract vaccine dose. Only 3 to 4 micrograms per gram of fresh leaf of E7 protein were produced and the quantity administered to the mice was 20-fold lower than that known to prevent tumor growth. The production levels of E7 by targeting it to the plant secretory pathway, which is used by cells to transport material to the outside. The researchers targeted the protein to the secretory pathway by adding so called signal sequences to the protein. The signal sequences guided the protein to specific areas for accumulation, for example to the endoplasmic reticulum, where the proteins may be retained or transported out of the cell. This offered a natural way to pre-concentrate the E7 protein.
The E7 production level was five-fold higher (15 micrograms of protein per gram of fresh leaf)compared to the production level in the cytoplasm.“This result may be related to a positive effect on protein stability,”In the endoplasmic reticulum,there are few enzymes that degrade proteins, but many so called chaperons that help proteins to fold correctly. An additional advantage of targeting E7 proteins to the secretory pathway is that the protein purification is further simplified, because the proteins might be naturally released from the roots or leaves of the plants.
Mice vaccinated with these plant extracts showed a stronger immune response and a more efficacious tumor protection than mice vaccinated with plant extracts producing the E7 protein in the cytoplasm. We believe that a further enhancement of this anti-tumor immunity can be achieved by a combination of E7-containing extracts with immunostimulatory genes, or by application of two different forms of the antigen.” The fact that freeze-dried N. benthamiana leaf tissues containing high amounts of E7 antigen are stable for at least one year at room temperature further improves production and storage of the vaccine. In addition, it offers the possibility to use such plant extracts as edible vaccines. “Edible plant based vaccines offer a palatable oral delivery system without the costly purification processes required for injectable vaccines. However, a great disadvantage of oral vaccine delivery is that the digestive system could degrade the antigens. This might limit the use of oral vaccines against pathogens that can survive in the harsh environment of the digestive system.
By using a plant system for antigen production, however, the vaccine antigens inside the plant cells are naturally protected by the cell wall “Edible vaccines are now considered to be more stable and practical in the harsh environment of the gastrointestinal tract than purified vaccine antigens.”
Oral immunization with tobacco plants, however, could raise the problem of toxicity, since many tobacco cultivars produce high levels of toxic alkaloids. Varieties with only low alkaloid amounts, however, have been shown to be safe production and delivery systems for therapeutic vaccines. Mice fed with such tobacco leaves showed no symptoms of toxicity.
A significant step toward the development of a vaccine against cancers caused by the Human Papillomavirus type 16. Since HPV16 is an enormous problem - particularly in the developing world - a vaccine against this virus needs to be highly effective, temperature-stabile and easy to administer. Furthermore, it should be possible to produce the vaccine on a large scale and cost-efficiently. Plants, as both a production and delivery system for vaccines, meet these requirements.
Enterotoxigenic E. Coli (humans)-TOBACCO - Immunogenic when administered orally
Enterotoxigenic E. coli{humans}- POTATO - Immunogenic and protective when administered orally
Enterotoxigenic E. Coli{humans}- MAIZE - Immunogenic and protective when administered orally
Vibrio cholerae [CHOLERA] (humans) - POTATO- Immunogenic and protective when administered orally
Hepatitis B virus {humans}- TOBACCO -Extracted protein is immunogenic when administered by injection.
Hepatitis B virus {humans} - POTATO - Immunogenic when administered orally
Hepatitis B virus {humans}- LUPIN - Immunogenic when administered orally
Hepatitis B virus {humans}- LETTUCE - Immunogenic when administered orally
Norwalk virus (humans) - TOBACCO - Immunogenic when administered orally
Norwalk virus (humans) - POTATO - Virus-like particles form and immunogenic when administered orally
RABIES virus (humans) -TOMATO - Intact Glycoprotein
A. Humanized Monoclonal Antibody Produced In Transgenic Plants For Immuno-Protection Of The Vagina Against Genital Herpes:(55)
The ability to produce monoclonal antibodies (Mabs) in plants offers the opportunity for the development of an inexpensive method of mucosol immuno protection against sexually transmitted diseases. To investigate the suitability of plant expressed Mabs for vaginal preventive applications, workers had compared a humanised anti herpes simplex virus 2 (HSV-2) Mab expressed in mammalian cell culture with the same antibody expressed in soyabean. They found these Mabs to be similar in their stability in human semen and cervical mucus over 24 hr., and their efficacy for prevention of vaginal HSV-2 infection in the mouse.
Compared with vaccine delivery by injection, oral vaccines offer the hope of more convenient immunization strategies and a more practical means of implementing universal vaccination programs throughout the world. Oral vaccines act by stimulating the immune system at effector sites (lymphoid tissue) located in the gut. Genetic engineering has been used with variable success to design living and non-living systems as a means to deliver antigens to these sites and to stimulate a desired immune response. More recently, plant biotechnology techniques have been used to create plants which contain a gene derived from a human pathogen; the resultant plant tissues will accumulate an antigenic protein encoded by the foreign DNA. In pre-clinical trials, workers found that antigenic proteins produced in transgenic plants retained immunogenic properties when purified; if injected into mice the antigen caused production of protein-specific antibodies. Moreover, in some experiments, if the plant tissues were simply fed to mice, a mucosal immune response occurred. The present study was conducted as a proof of principle to determine if humans would also develop a serum and/or mucosal immune response to an antigen delivered in an uncooked foodstuff.
Transgenic potatoes were engineered to synthesize a cholera toxin B subunit (CTB) pentamer with affinity for G sub (M1) - ganglioside. Both serum and intenstinal CTB-specific antibodies were induced in orally immunized mice. Mucosal antibody titers declined gradually after the last immunization but were restored following an oral booster of transgenic potato. The cytopathic effect of cholera holotoxin (CT) on Vero cells was neutralized by serum from mice immunized with transgenic potato tissues. Following intraileal injection with CT, the plant-immunized mice showed up to a 60% reduction in diarrheal fluid accumulation in the small intestine. Protection against CT was based on inhibition of enterotoxin binding to the cell-surface receptor G sub (M1) - ganglioside. These results demonstrate the ability of transgenic food plants to generate protective immunity in mice against a bacterial enterotoxin.
Researchers have engineered tomato plants (Lycopersicon esculentum Mill var. UC82b) to express a gene for the glycoprotein (G-protein), which coats the outer surface the rabies virus. The recombinant constructs contained the G-protein gene from the ERA strain of rabies virus, including the signal peptide, under the control of the 35S promoter of cauliflower mosaic virus. Plants were transformed by Agrobacterium tumefaciens-medicated transformation of cotyledons and tissue culture on selective media. PCR confirmed the presence of the G-protein gene in plants surviving selection. Northern blot analysis indicated that RNA of the appropriate molecular weight was produced in both leaves and fruit of the transgenic plants. The recombinant G-protein was immunoprecipitated and detected by Western blot from leaves and fruit using different antisera. The G-protein expressed in tomato appeared as two distinct bands with apparent molecular mass of 62 and 60 kDa as compared to the 66 kDa observed for G-protein from virus grown in BHK cells. Electron microscopy of leaf tissue using immunogold-labeling and antisera specific for rabies G-protein showed localization of the G-protein to the Golgi bodies, vesicles, plasmalemma and cell walls of vascular parenchyma cells. In light of previous demonstration that orally administered rabies G-protein from the same ERA strain elicits protective immunity in animals, these transgenic plants should provide a valuable tool for the development of edible oral vaccines.
The efficacy of edible vaccines produced in potato tubers was examined in mice. Transgenic plants were developed by Agrobacterium tumefaciens-medicated transformation. The antigen selected was the non-toxic B subunit of the Escherichia coli enterotoxin (recLT-B). A synthetic gene coding for recLT-B was made and optimised for expression in potato tubers and accumulation in the endoplasmic reticulum.
Introduction of this gene under control of the tuber-specific patatin promotor in potato plants resulted in the production of functional, i.e. Gm1-binding, recLT-B pentamers in tubers. Selected tubers containing about 13 µg of recLT-B per gram fresh weight were used for immunisation. Subcutaneous immunisation with an extract of recLT-B tubers yielded high antibody titres in serum that were similar to those obtained with bacterial recLT-B. The efficacy of oral administration of recLT-B tubers was determined by measuring mucosal and systemic immune responses in naive and primed mice. Animals were primed by subcutaneous injection of an extract of recLT-B tuber plus adjuvant. Naive and primed mice were fed 5 g of tubers ( ~ 65 µg of recLT-B) or were intubated intragastrically with 0.4 ml of tuber extract (~2 µg of recLT-B). In naive mice, feeding recLT-B tubers or intubation of tuber extract did not induce detectable anti-LT antibody titres. In primed animals, however, oral immunisation resulted in significant anti-LT lgA antibody responses in serum and faeces. Intragastric intubation of tuber extract revealed higher responses than feeding of tubers.
Advances in malaria vaccine and drug development have been hindered in part by the complex multistage life cycle of the parasite, much of which is inaccessible to study, and by a large genome encoding over 5000 genes. Two human models of immunity to malaria, however, suggest that the development of an effective vaccine is within reach. Scientists have outlined a strategy to identify the expression of hundreds to thousands of potential vaccine targets employing recently developed technologies for gene and protein expression. Combined with the exciting developments of malaria DNA vaccine technologies, these approaches form the basis for malaria subunit vaccines that may mimic the protective efficacy of our human model systems and provide the foundation for novel approaches to vaccine development for a range of pathogens.
Most edible vaccines are for gut pathogens. The question was raised as to whether the HPV VLPs would be stable enough to induce an immune response if inoculated via intragastric gavage. The following experiment was done in collaboration with Dr Bob Rose (Rochester University, NY), to investigate this possibility. Human papillomavirus type 11 (HPV-11) recombinant virus-like particles (VLPs) were produced in insect cells and evaluated for oral immunogenicity in BALB/c mice. When tested in an enzyme-linked immunosorbent assay (ELISA), sera from immunized animals demonstrated HPV-11 VLP-specific immunoglobulin G (IgG) and IgA responses that were dose-dependent, conformationally dependent, and genotype-restricted. Moreover, when tested in a newly developed VLP binding inhibition (VBI) enzyme-linked immunosorbent assay (ELISA), orally induced VLP antibodies were found to efficiently inhibit VLP binding by rabbit HPV-11 virus-neutralizing polyclonal antibodies. These results suggest that VLPs may be effective oral immunogens for the prevention of anogenital HPV disease.
Disease Target (Source of the Protein) |
Target Species for the vaccine plant expression system |
Notes and protective capacity of vaccine
|
Enterotoxigenic E. COLI (humans) |
TOBACCO |
Immunogenic when administered orally |
Enterotoxigenic E. COLI (humans) |
POTATO |
Immunogenic and protective when administered orally |
Enterotoxigenic E. COLI (humans) |
MAIZE |
Immunogenic and protective when administered orally |
Vibrio cholerae [CHOLERA] (humans) |
POTATO |
Immunogenic and protective when administered orally |
Hepatitis B virus {humans} |
TOBACCO |
Extracted protein is immunogenic when administered by injection |
Hepatitis B virus {humans} |
POTATO |
Immunogenic when administered orally |
Hepatitis B virus {humans} |
LUPIN |
Immunogenic when administered orally |
Hepatitis B virus {humans} |
LETTUCE |
Immunogenic when administered orally |
Norwalk virus (humans) |
TOBACCO |
Immunogenic when administered orally |
Norwalk virus (humans) |
POTATO |
Virus-like particles form and Immunogenic when administered orally |
RABBIES virus (humans) |
TOMATO |
Intact Glycoprotein |
Human cytomegalovirus {humans} |
TOBACCO |
Immunologically related protein |
Rabbit hemorrhagic disease virus {rabbits} |
POTATO |
Immunogenic and protective when administered by injection |
FOOT-AND-MOUTH disease {agricultural domestic animals} |
ARABIDOPSIS |
Immunogenic and protective when administered orally |
FOOT-AND-MOUTH disease {agricultural domestic animals} |
ALFALFA |
Immunogenic and protective when administered by injection or orally |
Transmissible gastroenteritis coronavirus (pigs) |
ARIBIDOPSIS |
Immunogenic when administered by injection |
Transmissible gastroenteritis coronavirus (pigs) |
TOBACCO |
Intact protein and Immunogenic when administered by injection |
Transmissible gastroenteritis coronavirus (pigs) |
MAIZE |
Protective when administered orally |
Currently researchers are seeking to develop genetically altered plants that could provide immunity to infectious diseases. Studies have already shown that genetically engineered plants can act as a vaccine. Plants acting as vaccines would offer the advantage of inexpensive to produce, and thus they could more easily be made available to developing countries. In addition, contamination with animal viruses would be eliminated, since cultured cells would not be used in the production process. Many of the quality control tests that require animals also could be eliminated.
Edible vaccines are currently being developed for a number of human and animal diseases, including measles, cholera, foot and mouth disease, and Hepatitis-B and C. Many of these diseases are likely to require booster vaccinations or multiple antigens to induce and maintain protective immunity. Plants have the capacity to express more than one transgene, allowing delivery of multiple antigens for repeated inoculations.
Recently the glare of the media spotlight has fallen on genetically engineered food crops bred to resist herbicides and insects. Meanwhile, plants engineered with human proteins to produce drugs and vaccines for human consumption have escaped notice. Well, take note: At least 350 genetically engineered pharmaceutical products are currently in clinical development in the United States and Canada . Scientists believe that potent drugs and vaccines will soon be harvested just like wheat and corn.
In Canada , a genetically engineered tobacco plant made to produce Interleukin 10 will be tested to treat Crohn's disease, an intestinal disorder.
Scientists have also discovered that fruits with high water content could result in proteolysis. Experimentation with freeze-dried food to create pellets or powder is now being investigated to help avoid proteolysis and overall efficacy.
Arntzen and his colleagues plan to develop banana-based vaccines. They're also targeting papilloma virus, which can lead to cervical cancer, as well as Hepatitis-B virus. A reliable injectable vaccine already exists for Hepatitis-B, but a less expensive, edible vaccine would be better, particularly for use in the developing world.
Current measles vaccines are made from the actual virus and work by priming the immune system to attack if it becomes exposed to a full assault of the measles virus. In contrast, plant-based vaccines rely on the measles virus gene for the H protein being genetically cloned into the plant.
Although still at an early stage of development, the experimental know-how and results strongly suggest that plant-derived edible vaccines are likely to become a reality, in the next few years. Future research will demonstrate if these vaccines meet the standards of quality (purity, potency, safety and efficacy) defined for vaccines by the World Health Organization.
In future a great ability for the immunization throughout the glob with the use of edible vaccine can be possible with its economic production will lower down the cost of immunization, separation and purification is easy and pathogenic contamination can be avoided therefore safe, no constricted criteria for its storage so can be stored near the site of use, aseptic condition dose not require for oral immunization as it is given through oral route.GM-plant (Genetically modified plants) may be grown in field and clinical trials are required to define the risk/ benefit ratio of a GM-plant before registration is granted.
In most countries of the world, plants engineered to produce vaccines fall under the very restrictive rules set up to control GM-crop plants. The present concern, especially in Europe , over the use of biotechnology for the genetic improvement of crop plants also negatively affects the acceptance of GM-plants for medicinal use. As a consequence, while the demonstration that plant-derived vaccines are effective on populations at risk is expected to arrive within 1-2 years, a further quarantine of 2-3 years will be required in order to fulfil requirements for registration and marketing. It is hoped that simpler rules will be set up for GM-plants producing vaccines and that they are seen as clearly and legally distinct from GM-plants grown for nutrition purposes.
Vaccines have been one of the most far-reaching and important public health initiatives of the 20th century. Advancing technology, such as oral DNA vaccines, intranasal delivery and edible plant derived vaccines, may lead to a future of safer and more effective immunization. Edible vaccines, in particular, might overcome some of the difficulties of production, distribution and delivery associated with traditional vaccines. Significant challenges are still to be overcome before vaccine crops can become a reality. However, while access to essential healthcare remains limited in much of the world and the scientific community is struggling with complex diseases such as HIV and malaria, plant derived vaccines represent an appetising prospect. The potential advantages of plant based vaccines are edible means of administration, reduced need for medical personnel and sterile injection conditions, economical to mass produce and transport, reduced dependence on foreign supply, storage near the site of use, heat stable, eliminating the need for refrigeration, antigen protection through bioencapsulation, subunit vaccine (not attenuated pathogens) means improved safety, seroconversion in the presence of maternal antibodies, generation of systemic and mucosal immunity, enhanced compliance (especially in children), delivery of multiple antigens, and integration with other vaccine approaches.
This review gives complete information about the importance of edible vaccines produced by transgenic plants. Transgenic plants expressing vaccine proteins are cost-effective, are easily reproduced, can package vaccine proteins in seed or fruit, and cannot be contaminated by mammalian pathogens. Multi-component vaccines could be produced through gene stacking. Plant tissues can be directly fed to patients as “edible vaccines”, which eliminates the need for purification and refrigeration, takes advantage of “bio-encapsulation”, and could stimulate mucosal immunity. Local agricultural production and processing facilities could be utilized.
This review provides all information from basics to protocol of production, newly developed edible vaccines specifically on HBV, Measles along with success stories. Hence the forthcoming era of this next generation vaccine may lead to a future of safer and more effective immunisation.
1. Arakawa, T., Chong, D.K.X., and Langridge, W.H.R. (1997). Expression of Choleratoxin B subunit oligomers in transgenic potato plants, Transgenic Res, 6: 403-413
2. Arakawa, T., Chong, D.K.X. and Langridge, W.H.R. (1998). Transgenic plants for the production of edible vaccine and antibodies for immunotherapy, Nature Biotechnol, 16: 292-297
3. Arakawa, T., Yu, J. and Chong, D.K.X. (1998). A plant based cholera toxin B subunit insulin fusion protein protects against the development of autoimmune diabetes, Nat. Biotechnol., 16: 934-938
4. Arntzen, C.J. (1998). Pharmaceutical foodstuffs-oral immunization with transgenic plants, Nature Medicine (supplement), 4: 502-03
5. Artsaenko, O., Peisker, M., Zurniedan, U., Fielder, U., Weiler, E.W., Muntz, K., Conrad, U. (1995). Expression of a single chain FV antibody against abscisic acid creats a wilty phenotypes in transgenic tobacco, Plant J., 8: 745-750
6. Baum, T.J., Hiatt, A., Parrott, W.A., Pratt, L.H., Hussey, R. S. (1996). Expression in tobacco of a monoclonal antibody specific to secretion of the root knot nematode, Mol. Plant Microbe Interact., 9: 382-387
7. Benvenuto, E., Ordas, R.J., Tavazza, R., Ancora, G., Biocca, S., Cattaneo, A. and Galeffi, P. (1991). Phytoantibodies: A general vector for the expression of immunoglobular domains in transgenic plants, Plant Mol. Biol., 17: 865-874
8. Carter, J.E.III and Langridge, W.H.R. (2004). Plant based vaccine for protection against infectious and autoimmune diseases, Crit. Rev. Plant Sci., 21
9.Lee RWH, Cornelisse M, Ziauddin A, Slack PJ, Hodgins DC, Strommer JN, Shewen PE, Lo RYC submitted. Expression of a derivative of Mannheimia haemolytica GS60 antigen, a member of the LppC family of bacterial outer membrane lipoproteins, in transgenic alfalfa for the development of an edible vaccine against bovine pneumonic pasteurellosis.
10.Lee RWH, Pool AN, Ziauddin A, Lo RYC, Shewen PE, Strommer JN 2003. Edible vaccine development: Stability of Mannheimia haemolytica A1 leukotoxin 50 during post-harvest processing and storage of field-grown transgenic white clover. Molec Breed 11: 259-266
11.answers.yahoo.com/question/index?qid=20070201051431AAGolMO&fr=oni_o.nnetwork_se_aglbl3 years ago
12. clasdean.la.asu.edu/news/arntzen.htm
13. iptv.org/exploremore/ge/features/bananavaccine.cfm
14. nal.usda.gov/pgdic/Probe/v5n1/lead.html
15. oru.se/templates/oruExtNormal__40417.aspx
16. molecularfarming.com/ediblevaccine.html
17. abc.net.au/science/articles/1998/11/17/17094.htm
18. facstaff.uwa.edu/dsalter/cell%20b
19. answers.yahoo.com/question/index?qid=20070201051431AAGolMO&fr=oni_onnetwork_se_aglbl3 years ago
20. hip2b2.com/news/killer-tomatoes-attack-human-diseases/85938/
21. A Review of the Progression of Transgenic Plants Used to Produce Plantibodies For Human Usage, Journal of Young Investigators. 2001, Volume IV
22. Measles (Care and treatment), Biological products industry (Research), Genetically modified plants (Research), Vaccines (Research), Vaccines for Human Use
Published By: The Futurist, Sept 2001, United States.
23. Biotechnolgy, By- Dr. U. Satyanarayanan, Books & allied (P) Ltd, pg no. 572-573.
24. Foods as Production and Delivery Vehicles for Human Vaccines, Journal of the AmericanCollege of Nutrition, Vol. 21, No. 3, 212S–217S (2002)
25. Thanavala, Y F Yang, P Lyons, H S Mason, and C Arntzen, Immunogenicity of transgenic plant-derived hepatitis B surface antigen, Proc Natl Acad Sci U S A. 1995 April 11; 92(8): 3358–3361
26. Alexander V. Karasev, Scott Foulke, Candice Wellens, Amy Rich, Kyu J. Shon, Izabela Zwierzynski, David Hone, Hilary Koprowski and Marvin Reitz, Plant based HIV-1 vaccine candidate: Tat protein produced in spinachVaccine, Volume 23, Issue 15, 7 March 2005, Pages 1875-1880
27. Diane E Webster, Merlin C Thomas, Richard A Strugnell, Ian B Dry and Steve L Wesselingh, Appetising solutions: an edible vaccine for measles, The Medical Journal of Australia, MJA 2002; 176 (9): 434-437
28. Summary of Invited Plant-derived Vaccines: A New Approach to International Public Health White Paper and Responses An IUFoST Internet Forum By Pamela Tom1 and Daryl Lund
29. Mahender Singh Rathore, A Review of the plant Derived Vaccines, www.geocities.com/plantvaccines, published: Feb.15, 2004
30. Hiatt, A., Cafferkey, R. and Bowdish, K., Nature, 1989, 342, 76–78.
31. Mason, H. S. and Arntzen, C. J., Trends Biotechnol., 1995, 13, 388–392.
32. Mason, H. S., Lam, D. M. and Arntzen, C. J., Proc. Natl. Acad. Sci. USA, 1992, 89, 11745–11749.
33. De Block, M., Herrera-Estrella, L., van Montagu, M., Schell, J. and Zambryski, P., EMBO J., 1984, 3, 1681–1689.
34. Birch, R. G., Annu. Rev. Plant Physiol. Mol. Biol., 1997, 48, 297–326.
35. Cramer, C. L., Weissenborn, D. L., Oishi, K. K., Grabau, E. A., Bennett, S., Ponce, E., Grabowski, G. A. and Radin, D. N., Ann. NY Acad. Sci., 1996, 792, 62–71.
36. Ma., J. K. and Hein, M. B., Trends Biotechnol., 1995, 13, 522–527.
37. Christou, P., Plant Mol. Biol., 1997, 35, 197–203.
38. McElroy, D. and Brettell, R. I. S., Trends Biotechnol., 1994, 12, 62–68.
39. Tyagi, A. K., Bajaj, S., Mohanty, A., Chaudhury, A. and Maheshwari, S. C., Crit. Rev. Biotechnol., 1999, 19, 41–79.
40. Schledzewski, K. and Mendel, R. R., Transgenic Res., 1994, 3, 249–255.
41. Haq, T. A., Mason, H. S., Clements, J. D. and Artnzen, C. J., Science, 1995, 268, 714–715.
42. Chrispeels, M. J. and Tague, B. W., Int. Rev. Cytol., 1991, 125, 1–45.
43. Lund, P. and Dunsmuir, P., Plant Mol. Biol., 1992, 18, 47–53.
44. Thanavala, Y., Yang, Y.-F., Lyons, P., Mason, H. S. and Arntzen, C., Proc. Natl. Aad. Sci. USA, 1995, 92, 3358–3361.
45. Mason, H. S., Ball, J. M., Shi, J. J., Jiang, X., Estes, M. K. and Arntzen, C. J., Proc. Natl. Acad. Sci. USA, 1996, 93, 5335–5340.
46. Mason, H. S., Haq, T. A., Clements, J. D. and Arntzen, C. J., Vaccine, 1998, 16, 1336–1343.
47. Hein, M. B., Yeo, T.-C., Wang, F. and Sturtevant, A., Ann. NY Acad. Sci., 1996, 792, 51–56.
48. Arakawa, T., Chong, D. K. X., Merritt, J. L. and Langridge, W. H. R., Transgenic Res., 1997, 6, 403–413.
49. Arakawa, T., Chong, D. K. X. and Langridge, W. H. R., Nature Biotechnol., 1998, 16, 292–297.
50. Ma, S.-W., Zhao, D. L., Yin, Z. Q., Mukherjee, R., Singh, B., Quin, H. Y., Stiller, C. R. and Jevnikar, A. M., Nature Med., 1997, 3, 793–796.