{ DOWNLOAD AS PDF }
ABOUT AUTHOR
Komal Saini*
Department of Pharmaceutics, University Institute of Pharmaceutical Sciences, Panjab University, Chandigarh
komalsainiks@gmail.com
ABSTRACT
Hydrogels comprises of different classes class of materials that could absorb considerable amount of water while maintaining their integrity in water having three dimensional, hydrophilic, polymeric networks. In the last few years, radical methods of preparation of hydrophilic polymers and hydrogels have evolved that may be used in the future in drug delivery applications. For successful and desirable applications, synthesis of new polymers and cross-linkers having more biocompatibility and better biodegradability would be beneficial. This review provides an overview of the different classes of hydrogels, their characterization, method of preparation, their advantages and disadvantages and cross-linking.
REFERENCE ID: PHARMATUTOR-ART-2454
PharmaTutor (ISSN: 2347 - 7881) Volume 5, Issue 1 Received On: 25/07/2016; Accepted On: 29/08/2016; Published On: 01/01/2017 How to cite this article: Saini K; Preparation method, Properties and Crosslinking of hydrogel: a review; PharmaTutor; 2016; 5(1); 27-36 |
INTRODUCTION
Polymer hydrogels are rapidly developing group of materials, providing wide application in many fields, especially pharmacy, medicine and agriculture. Many reports have been published about new chemical and physical structures, properties and innovative restricted applications of polymer hydrogels. They are three-dimensional, hydrophilic, polymeric networks having swelling property and hold a large amount of water while maintaining the structure. Their ability to absorb water is due to its crosslinking network structure which is developed by polymer bearing hydrophilic groups such as –OH, –CONH, –COOH, –SO3H, and –NH21 .
There are different types of macromolecular structures that are possible for physical and chemical hydrogels. They includes cross-linked or entangled networks of linear homopolymers, linear copolymers, and block or graft copolymers; polyion–multivalent ion, polyion–polyion or H–bonded complexes; hydrophilic networks stabilized by hydrophobic domains; and IPNs or physical blends. Hydrogels may also have different types of physical forms2 given in table 1.
Table 1: Different physical forms of hydrogel
Physical forms |
Examples |
1. Solid molded forms |
e.g., soft contact lenses |
2. Pressed powder matrices |
e.g., pills or capsules for oral ingestion |
3. Microparticles |
e.g., as bioadhesive carriers or wound treatments |
4. Coatings |
e.g., on implants or catheters; on pills or capsules; or coatings on the inside capillary wall in capillary electrophoresis |
5. Membranes or sheets |
e.g., as a reservoir in a transdermal drug delivery patch; or for 2D electrophoresis gels |
6. Encapsulated solids |
e.g., in osmotic pumps |
7. Liquids |
e.g., that form gels on heating or cooling |
Among the numerous polymers that have been proposed for the preparation of hydrogels, polysaccharides have a number of advantages over the synthetic polymers which were initially employed in the field of pharmaceutics. Indeed, polysaccharides are abundant and obtained from renewable sources such as the algal and plant kingdoms, cultures of microbial selected strains, as well as through recombinant DNA techniques. They can be formulated or synthesized from natural or synthetic polymers. Although hydrogels made from natural polymers may not provide sufficient mechanical properties and may contain pathogens or evoke immune/inflammatory responses, provides several advantageous properties such as inherent biocompatibility, biodegradability, and biologically recognizable moieties that support cellular activities. Synthetic hydrogels do not show these inherent biological properties. Fortunately, synthetic polymers usually have well-defined structures that can be modified to yield the tailorable degradability and functionality. The specific application of plant-derived polymers in pharmaceutical formulations include their use in the manufacture of solid monolithic matrix systems, implants, films, beads, microparticles, nanoparticles, inhalable and injectable systems as well as viscous liquid formulations3. Table 1 shows the natural polymers as well as synthetic monomers that are commonly used in hydrogel fabrication is given below:
Table 2: List of natural and synthetic polymers
Natural Polymers |
Synthetic Polymers |
1.Alginate 2.Dextran 3.Guar gum 4.Xanthan 5.Hyaluronic acid 6.Pullulan 7.Xyloglucan 8.Gellan 9.Scleroglucan 10. Pectin 11. Okra gum 12. Locust gum |
1.Poly (vinylalcohol) 2.Acrylic acid (AA) 3.Methacrylic acid (MAA) 4.poly (styrene) (PS) 5.poly (N-vinylpyrrolidone) (PVP) 6.Polyethyleneglycol acrylate/methacrylate (PEGA/PEGMA) 7.Polyethyleneglycol diacrylate/dimethacrylate (PEGDA/PEGDMA) 8.chitosan |
For over fifty years, hydrogels have been utilized in several biomedical disciplines, in ophthalmology as contact lenses as well as in vast areas of clinical practice to heal such illnesses as diabetes mellitus, osteoporosis, asthma, heart diseases and neoplasms. The first hydrogel that was synthetic poly-2-hydroxyethyl methacrylate has been synthsized with biomedical applications and used in contact lens production. The main advantage of that potential biomaterial was its stability under varying pH, temperature and tonicity conditions. Hydrogels were also modified and characterized for some other applications. Lim and Sun obtained calcium alginate microcapsules for cell engineering, and Yannas’ group modified synthetic hydrogels with some natural substances, such as collagen and shark cartilage to obtain novel dressings, providing optimal conditions for healing burns. The final hydrogel product is present in tissue engineering and regeneration and it can be used to cure the thrombosis, post-operative adhesion formation, drug delivery systems, coatings for biosensors and cell transplantation4.
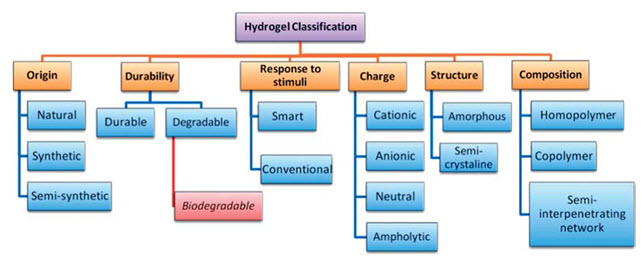
Various classifications have been employed to hydrogels as depicted in Fig. 1. Some of these classification5 are given below:
1. According to origin: - Hydrogels can be classified into natural, synthetic and semi-synthetic on the basis of their origin. Most of the synthetic hydrogels are synthesized by traditional polymerization of vinyl or vinyl-activated monomers. The equilibrium swelling values of these synthetic hydrogels vary broadly due to their hydrophilicity of the monomers and the crosslinking density. An in situ crosslinking reaction is carried out by using a bi-functional monomer. Natural hydrogels comprised of natural polymers including polynucleotides, polypeptides, and polysaccharides. These natural polymers are obtained from various natural origins like plants, animals, or marine sources.
2. According to hydrogel durability: - Hydrogels can be either durable (such as most polyacrylate-based hydrogels) or biodegradable (such as polysaccharides-based hydrogels), due to their stability in a physiological environment. Biodegradation of polymeric biomaterials including the breakdown of hydrolytically or enzymatically sensitive bonds leading to polymer erosion6. Based on the nature of degradation, polymeric biomaterials can be further classified into hydrolytically degradable polymers and enzymatically degradable polymers. Biodegradable hydrogels shows the both biomedical and non-biomedical uses. The degradable polymers inside the hydrogel matrices undergo chain fission to form oligomers having low molecular weight. Then, the resulting oligomers are either eliminated by body or undergo further degradation.
3. According to hydrogel response to environmental stimuli:- Hydrogels can also control drug release by changing the gel structure in response to environmental stimuli. Hydrogels containing such ‘sensor’ features can undergo reversible volume phase transitions or gel–sol phase transitions upon only very small changes in the environmental condition. This type of environment sensitive hydrogels is also called ‘Intelligent’ or ‘smart’ hydrogels. Many physical and chemical stimuli have been employed to induce various responses in the smart hydrogel systems. The physical stimuli consist of temperature, electric fields, solvent composition, light, pressure, sound and magnetic fields, while the chemical or biochemical stimuli consist of pH, ions and specific molecular recognition events. Smart hydrogels have been used in assorted applications, such as in making artificial muscles, chemical valves, immobilization of enzymes and cells, and concentrating dilute solutions in bioseparation. Environment-sensitive hydrogels are potential candidate for developing self-regulated drug delivery systems.
4. According to type of ionic charges present on polymer networksIonic hydrogels: - Ionic hydrogels contain charged anionic or cationic monomeric species. These may be homopolymeric involving only ionic polymer network or copolymer of ionic and neutral polymer network. They may be developed by modifications in neutral hydrogels by partial hydrolysis or addition of excess of polyelectrolytes. For eg. Anionic hydrogels (anionic thermo-associative carboxymethylpullulan hydrogels) and Cationic hydrogels (new thermosensitive, cationic hydrogels of N-isopropylacrylamide (NIPAM) and (3-acrylamidopropyl) trimethylammonium chloride (AAPTAC)7.
Neutral hydrogels: - These hydrogels are temperature sensitive resulting in swelling–deswelling behavior with change in temperature having permanent polymer networks as linkages formed are irreversible. This kind of linkage allows absorption of water without dissolution and thus allows drug release by diffusion. Neutral hydrogels (miscible blends from water-insoluble polymers like poly (2,4,4-trimethylhexamethylene terephthalamide).
Ampholytic hydrogels: - Ampholytic hydrogels have polymeric networks having both positively and negatively charged monomeric species. The properties of ampholytic hydrogels depend upon the presence of ionic species along the polymer chain. Oppositely charged solutions have coulombic attractions between them due to which ampholytic hydrogels have interionic as well as intra-ionic attractions for eg. acrylamide based ampholytic hydrogels8.
NOW YOU CAN ALSO PUBLISH YOUR ARTICLE ONLINE.
SUBMIT YOUR ARTICLE/PROJECT AT editor-in-chief@pharmatutor.org
Subscribe to Pharmatutor Alerts by Email
FIND OUT MORE ARTICLES AT OUR DATABASE
Properties of hydrogels
Hydrogels plays an important role in the field of pharmaceutical and biomedical engineering. It can be used as a carrier for drug and other therapeutic bio-molecule. These biomaterials evaluate the characteristic properties like swelling behavior, mechanical properties and toxicity studies etc so that the hydrogel could be used successfully in the concerned biomedical field.
1. Swelling properties
All hydrogels polymer chains are cross linked to each other physically as well as chemically. There is no subject of molecular weight of hydrogels and therefore, sometimes called super macromolecules. A small change in environmental condition may elicit fast and reversible changes in hydrogel. The fluctuations in environmental parameters like pH, temperature, electric signal, presence of enzyme or other ionic species may lead to vary physical texture of the hydrogel. These changes may arise at macroscopic level as precipitate formation, changes in size and water content of hydrogels. Hydrogels with acidic or basic functional groups rise to the fluctuations in the external environmental pH. Polyacrylic acid is such type of pH sensitive hydrogel where swelling ratio changes attributed to the ionization of carboxyl groups on the polymer chain9.
Temperature-induced phase transitions and microenvironment of PNIPAM based hydrogels were studied in water, using 9-(4-N,N-dimethylaminophenyl) phenanthrene (DP) as an intramolecular fluorescence probe. Fluorescence behaviors of the DP-labeled PNIPAM gels depend on the concentrations of monomer and cross-linker. Thermo-responsive behavior of the PNIPAM hydrogel was influenced by copolymerization of NIPAM with a hydrophilic monomer N, N-dimethylacrylamide (DMAM) and a hydrophobic monomer methyl methacrylate (MMA). Incorporation of DMAM promoted the lower critical solution temperature (LCST) of the PNIPAM hydrogel and MMA reduced it. The results indicate that the NIPAM-DMAM copolymer hydrogel with elevated LCST are more open with water-swollen nature over their LCST while the NIPAMMMA co-polymer hydrogels with poorer LCST are less open beside water shrunken nature below their LCST when compared with PNIPAM homo-polymer hydrogel.
The swelling kinetics of hydrogels can be divided into diffusion-controlled (Fickian) and relaxation-controlled (non-Fickian) swelling kinetics. When water diffusion into the hydrogel occurs much faster than the relaxation of the polymer chains, the swelling kinetics is diffusion-controlled. The crosslinking ratio i.e. the ratio of moles of crosslinking agent and moles of polymer repeating units, is one of the best factor that influence the swelling of hydrogels. The higher the crosslinking ratio, the more crosslinking agent is integrated in the hydrogel structure. Highly crosslinked hydrogels have a compacted structure, and will swell in small amount as compared to the same hydrogels with lower crosslinking ratios. Crosslinking obstructs the mobility of the polymer chain, hence decreasing the swelling ratio. The swelling ratio of the hydrogels may also influenced by chemical structure of polymer.
Experimentally, % age swelling can be determined by weight difference method and is represented by the following equation10
% S=Ws-Wd/Wd*100
Where, Ws = weight of swollen gel and Wd = weight of dry gel
2. Mechanical properties
It is important to illustrate the hydrogels for their mechanical properties. Due to this property, hydrogel shows the various biomedical applications, viz. ligament and tendon repair, wound dressing material, matrix for drug delivery and tissue engineering, and as cartilage replacement, which requires hydrogels with different properties. Drug delivery is a method of administering a pharmaceutical compound to obtain a therapeutic effect in or at a certain location in the human body. Materials used as drug delivery systems need to have controlled properties, such as absorption and release profile and non toxicity.
As for example, a drug delivery device should retain its reliability during the lifetime of the application. A common method of increasing the mechanical strength is by increasing the crosslinking density, resulting in the formation of stronger gels. But with the rise in crosslinking density there is also lowering the % elongation of the hydrogels i.e. the hydrogels turn into brittle nature. Thus, an optimum degree of crosslinking depending on the desired properties of the final products. Co-monomer is copolymerized with increase in H-bonding within the hydrogel, has also been utilized by many researchers to attain preferred mechanical properties.
Grassi et al. determined the mechanical properties of calcium alginate hydrogel. The mechanical characterization consisted of the relaxation experiments (normal stress relaxation at constant deformation) to determine the hydrogel linear viscoelastic range and to describe the relaxation spectra and Young modulus by using the generalized Maxwell model. On the basis of Young modulus and Flory’s theory, hydrogels crosslinking density has also been determined. This value was then employed to calculate the average polymeric mesh size according to the equivalent network theory11.
3) Biocompatible properties
It is essential for the hydrogels to be biocompatible and nontoxic in order to make it remarkable parameter in biomedical field. Mostly polymers used for this concept must follow the cytotoxicity and in-vivo toxicity tests. Biocompatibility is the capability of a material to attain with an appropriate host response in a definite application. Biocompatibility consists basically of two elements: (a) bio-safety i.e. appropriate host response is systemic as well as local (the surrounding tissue), the absence of cytotoxicity, mutagenesis, and/or carcinogenesis and (b) bio-functionality i.e. the ability of material to carry out the specific task for which it is proposed.
In vitro tests for biocompatibility generally looks into the cyto-toxicity feature of the material in the presence of live host cells and can usually be performed in two ways. In the first method, the material whose biocompatibility has to be determined is positioned in direct contact with the host environmental cells and is afterward incubated for a specific period of time at 37°C. In the second method, the material is positioned in a suitable physiological solution and is then incubated for a specified period of time at 37°C to permit any leaching from the material. The obtained leachates are used to accomplish the biocompatibility tests in the presence of the cells. The researchers usually carry out the cell viability and cell proliferation from the cyto-toxicity test. The cell proliferation can be visualized by microscopy as well as by carrying out MTT (tetrazolium salt, 3-[4, 5-dimethylthiazol-2-yl]-2, 5-diphenyl tetrazolium bromide) assay i.e. a colorimetric method which permits quantification of cell growth and proliferation12.
Polymerization of synthetic hydrogels having toxic chemicals presents a challenge for in vivo biocompatibility if conversion is not 100%. In addition, initiators, organic solvents, stabilizers, emulsifiers, unreacted monomers and cross-linkers used in polymerization and host cells may be toxified by hydrogel synthesis if they escape to tissues or encapsulated cells. For example, Irgacure 2959, a typical photo-initiator used in several free radical photo-polymerizations. Though natural polymers are repeatedly considered to have greater biocompatibility over synthetic polymers, yet the continuation of synthetic cross-linkers and initiators used in the polymerizations of naturally derived monomers and pre-polymers are subject to the same toxicity concerns as purely synthetic hydrogels.
4. Water vapor transmission rate (WVTR):- It is the quantity of water vapor under precised temperature and humidity conditions, which passes through unit area of film material in predetermined time. WVTR is measured in grams per square meter (g/m2) over a 24 hours period according to the US standard ASTM E96-95. It is inversely related to the moisture retentive nature of a wound dressing i.e. the wound dressing with lower WVTR will be capable to maintain wound surface moisture. Usually, a wound dressing material showing WVTR less than 35 g/m2/hr is defined as moisture retentive and helps in a fast curing13.
Preparation methods of hydrogel
Hydrogels can be prepared by different methods depending on the designed structure and the preferred application. General method of hydrogel formation is given in fig. 1. Some of these methods are discussed below.
Fig. 2: Hydrogel preparation block diagram
Free radical polymerization
The ideal method conventional free radical polymerization is used for preparation of hydrogels based on various monomers such as acrylates, amides and vinyl lactams. It can also be used for development of natural polymers-based hydrogels having appropriate functional groups or have been functionalized with radically polymerizable groups. For example, chitosan-based hydrogels14. This method involves the typical free radical polymerization steps; initiation, propagation, chain transfer and termination. In the initiation step a wide variety of visible, thermal, ultraviolet and red-ox initiators can be used for radical generation. These radicals then react with monomers converting them into active forms which react with further more monomers and so on in the propagation step. The resulting long chain radicals undergo termination either through chain transfer or through radical combination developing polymeric matrices. This method of hydrogel preparation can be expressed either in solution or neat (bulk). Solution polymerization is advantageous during production of large quantities of hydrogels and in this case, water is the most commonly used solvent. Bulk polymerization, however is faster than solution polymerization and does not need a solvent removal, which is sustained in many cases.
Photopolymerization is one of the important process that enables in situ formation of crosslinked networks at physiological pH and temperature. The mild gelation conditions permit for cells to be encapsulated within photocrosslinked hydrogels and stay viable. The unique benefit of chain polymerization is the alleviate with which a variety of chemistries can be integrated into the hydrogel by simply mixing derivatized macromers of choice and afterward copolymerizing. Additionally, photopolymerized hydrogel systems can afford better temporal control over the gelation process, and they are injectable in nature, and can polymerize in situ to fill defects of any shape. Many researchers are interested in utilizing the photoinitiated polymerization of PEG-based macromolecular monomers to produce hydrogels as cell delivery vehicles for tissue regeneration. A comprehensively studied PEG hydrogel system is to occupy ultraviolet irradiation to generate radicals from specific photoinitiators, which will further react with the active end group on tailored PEG to form a covalent crosslinked bond. For cell delivery processes, PEG is comprised of a biochemically inert polymer that has no ability to adhere cells. Additionally to PEG, alginate, chitosan, hyaluronic acid and chondroitin sulfate were also methacrylated and hydrogels were synthesized by photopolymerization and other free radical polymerizations15.
Irradiation crosslinking
Ionizing-radiation techniques, mainly if combined with a simultaneous sterilization process, are very precious methods for preparation of hydrogels. High energy radiation, such as gamma and electron beam radiation can be employed to polymerize unsaturated compounds. Water soluble polymers functionalized with vinyl groups can be transformed into hydrogels using high energy radiation. Ionizing radiations, such as electron beam and g–rays have high energy as much as necessary to ionize simple molecules either in air or water16. During irradiation of a polymer solution, many reactive sites are generated alongside the polymer strands. After that, the combination of these radicals leads to formation of a large number of crosslinks. However, irradiation of a polymer solution is supported due to the less energy entailed for formation of macroradicals. Moreover, in solution the efficiency of radicals is high due to the reduced viscosity of reaction mixture.
Applying irradiation to hydrogel development offers the advantages over other preparation methods where no catalysts or additives are required to initiate the reaction. Also, irradiation methods are simple and the crosslinking point can be controlled easily by differing the irradiation dose. This technique has been used to formulate the wide range of hydrogels for many biomedical applications. For example, it has been used efficiently to synthesized acrylic acid hydrogels and poly (ethylene glycol)/carboxymethyl chitosan-based pH-responsive hydrogels. However, this method is not suggested for preparation of hydrogels from some polymers that can disgrace under the ionizing irradiation.
Chemical crosslinking
Chemical crosslinking is one of the major technique of hydrogel synthesis. In this method, a bi-functional crosslinking agent is added to a dilute solution of a hydrophilic polymer and the polymer must have an appropriate functionality to react with the crosslinking agent. This method is applicable for preparation of hydrogels from natural as well as synthetic hydrophilic polymers. For example, albumin and gelatin–based hydrogels were prepared via dialdehyde or formaldehyde as crosslinking agents. Hydrogels of high water content undergo crosslinking of functionalized polyethylene glycol and a lysine-containing polypeptide have been prepared by this method.
Chemically crosslinked hydrogels are developed by chain growth polymerization, addition and condensation polymerization and gamma and electron beam polymerization techniques. Addition and condensation polymerization includes a stepwise addition of polyfunctional crosslinking agents having monomer functional groups. Water soluble monomers can be translated into hydrogels using crosslinking agents such as tetramethylethylenediamine (TEMED). Polymer chains possibly crosslinked to form a hydrogel in the presence of water. Water occupies voids in the network, giving the hydrogel its distinctive surface properties. Polyurethanes, polyesters, or nylon polymers are normally prepared for hydrogel applications17.
Copolymerization/Crosslinking Reactions
Copolymerization reactions are applied to produce polymer gels, many hydrogels are produced in this concept, for example poly (hydroxyalkyl methylacrylates).
Cross-linking with aldehydes
For establishment of cross-linking, fixed conditions are applied like low pH, methanol added as a quencher, high temperature. Hydrophilic polymers with –OH groups e.g. polyvinyl alcohol may be cross-linked via glutaraldehyde. Alternatively, polymers with the amine-groups may be crosslinked with the same cross-linker of mild conditions in which Schiff bases are formed. It was especially proposed for the cross-linked protein synthesis, for instance, gelatin and albumin, and the amine enclosing polysaccharides.
Crosslinking Using Enzymes: A new method has been reported using an enzyme to formulate PEG-based hydrogels. A tetrahydroxy PEG was derivatized with addition of glutaminyl groups and networks were appeared by accumulation of transglutaminase into solution of PEG and poly (lysine-cophenylalanine). This enzyme catalyzes the reaction between the γ-carboxamide group of the PEG-Qa and the ε-amine group of lysine to give an amide linkage involving the polymers. The gel properties may possibly customized by the ratio of the PEG-Qa and the lysine copolymer. Under appropriate conditions, gels have been prepared with an equilibrium water content of 90%.
Sperinde et al. substituted the poly (lysine-co-phenylalanine) by lysine end-functionalized PEG18 and hydrogels were produced once transglutaminase was put into an aqueous solution of peptide modified macromers. The gelation kinetics undergoes macromer structure and composition, the ratio of the reactants and the enzyme concentration.
Transglutaminases are Ca2+-dependent enzymes. Depend here on, Westhaus et al. intended a triggered gelling system of hydrogels. A mixture of Ca-loaded liposomes, fibrinogen and a Ca2+-dependent transglutaminas sustained fluid when kept at room temperature, but gelled quickly when heated to 37 °C. Because of heat-treatment, the liposomes were destabilized and Ca-ions were liberated in the surrounding fluid simultaneously activating the enzyme. Due to this concept, this system is established at room temperature and solidifies at 37 °C, the authors suggest that this gel system can be employed as a matrix for the bioactive substances delivery for instance to promote tissue repair.
Physical Crosslinking of Hydrogels
Physically crosslinked hydrogels are developed by ionic interaction, crystallization, stereocomplex formation, hydrophobized polysaccharides, protein interaction and hydrogen bond.
Crosslinking by ionic interactions
In ionic interactions, hydrogels can be crosslinked under gently conditions, at room temperature and physiological pH. This process of cross-linking does not necessitates presence of ionic groups in the polymer. Metallic ions produce stronger hydrogel. Alginate is illustrious example of a polymer that can be crosslinked by ionic interactions.
Alginate is a natural polysaccharide having mannuronic and glucuronic acid remains and calcium ions crosslinking. Crosslinking can be observed at room temperature and physiological pH. Therefore, alginate gels are repeatedly employed as matrix for the encapsulation of living cells and for proteins release. Captivatingly, the gels can be destabilized by extraction of the Ca-ions from the gel via chelating agent. The release of proteins from alginate microparticles, achieved by spraying a solution of sodium alginate into an aqueous solution of calcium chloride, can be transformed by coating the particles having cationic polymers, for instance, chitosan and the other is polylysine.
A synthetic polymer, poly-[di(carboxylatophenoxy)phosphazene (PCPP) can also be crosslinked with Ca-ions. Gel microbeads were formed by spraying an aqueous solution of PCPP to an aqueous solution of calcium chloride. The ionotropic hydrogels disgrace under physiological conditions. Polycations can be crosslinked with anions. Chitosan is a biopolymer comprised of β-(1-4)-linked glucosamine units and is attained by deacetylation of chitin.
Chitosan-based hydrogels were attained by crosslinking of this polymer with glycerol-phosphate disodium salt19. Captivatingly, in the presence of this salt, chitosan solutions maintain liquid below room temperature, but promptly becomes a gel under heating. The temperature at which a sol–gel transition arises decreased with increasing degree of deacetylation. Carrageenan is a natural polysaccharide, composed of 1,4-linked α-D-galactose and 1,3 linked-β-D-galactose with a erratic part of sulfate groups, forms a gel with e.g. potassium ions, but also demonstrates gelation under salt-free conditions. But the gels were prepared in the presence of metallic ions and considerably stronger than those obtained under salt-free conditions. Besides to anionic polymers being crosslinked with metallic ions, hydrogels can also be attained by complexation of polyanions with polycations. Ionically crosslinked chitosan hydrogels are produced via complex formation of chitosan and polyanions, like dextran sulfate or polyphosphoric acid. Doxorubicin was entraped in nanoparticles of the chitosan hydrogels. These particles demonstrated a minimal burst release and kept good in-vitro cytotoxicity attributed to release drug.
Crosslinking by crystallization
Crystallization involves freezing/thawing process and creates a strong and highly elastic gel. PVA hydrogels can be formed by physically crosslinking through repeated freezing/thawing methods, or chemically crosslinked with glutaraldehyde or epichlorohydrin. Poly (vinyl alcohol) (PVA) is a water-soluble polymer. When aqueous solutions of PVA are stored at room temperature they progressively form a gel with, however, a low mechanical strength. Captivatingly, once aqueous solutions of this polymer undertake a freeze–thawing process a strong and enormously elastic gel is produced.
The properties of the gel depend on the PVA molecular weight, the PVA concentration in water, the temperature and time of freezing and the number of freezing cycles. Gel formation is attributed to the arrangement of PVA crystallites which acts like a physical crosslinking sites in the network. Gels formed under optimized conditions were secure for 6 months at 37 °C. PVA gels containing BSA were basically achieved by dissolving the protein in the aqueous PVA solution followed by freeze–thawing cycles. BSA was released by Fickian diffusion and with preservation of its conformation. The gel characteristics based on the amount of polymer present in the solution. Mainly microspheres were obtained by stirring. Gel formation is most likely caused by crystallization attributed to association of chains through hydrogen bonding.
Crosslinking by stereocomplex formation
For stereocomplex formation, a hydrogel is created through crosslinking that is produced between lactic acid oligomers of reverse chirality. The homopolymers of L-lactic acid and D-lactic acid are PLLA and PDLA (semi-crystalline substances) respectively. High molecular weight PLLA or PDLA, of either stereoisomer, has 170°C Tm (melting temperature) but in blending of high molecular weight PLLA and PDLA, a 230 ºC Tm is observed, which is due to the stereocomplex formation. Protein-loaded hydrogels were basically formed by suspending the protein in the dextran-g-oligolactate solutions prior to mixing. It was revealed that with the physiological conditions, the gels are entirely degradable. The degradation time based on the composition of the hydrogel, i.e. the number of lactate grafts, the length and polydispersity of the grafts and the initial water content, and changed from 1 to 7 days. The gels revealed a release of the encapsulated model proteins (IgG and lysozyme) over 6 days and the kinetics based on the gel individuality, such as the polydispersity of the lactate grafts and the initial water content. Significantly, the proteins were quantitatively released from the gels and with entire preservation of the enzymatic activity of lysozyme, highlight the protein-responsive preparation method of the protein-loaded stereocomplex hydrogel20.
Crosslinking by hydrogen bonding
Hydrogen bonding between polymer chains can also participate in hydrogel formation like in developing gelatin-based hydrogel. A hydrogen bond is produced through the involvement of an electron deficient hydrogen atom and a functional group of high electronegativity. Hydrogels extended by this technique are influenced by various factors like polymer concentration, molar ratio of each polymer, type of solvent, solution temperature, and the degree of association of polymer functionalities.
Advantages of hydrogel
Environmentally sensitive hydrogels shows the ability to logic changes of pH, temperature, or the concentration of metabolite and release their load as outcome of such a change. Hydrogel is stronger and more flexible. Encapsulation of microbial cells within polyurethane hydrogel beads allows the advantage of low toxicity. Natural hydrogel materials are being examined for tissue engineering, these materials incorporate agarose, methylcellulose, hyaluronan, and other naturally derived polymers. Hydrogel-based microvalves shows a number of advantages over conventional microvalves, incorporating moderately simple fabrication, no outer power requirement, no integrated electronics, large displacement and large force generation.
Disadvantages
The main disadvantages are the high cost and the sensation felt by movement of the maggots. It involves thrombosis at anastomosis sites and the surgical risk associated with the device implantation and retrieval. Hydrogels are nonadherent; they may require to be protected by a secondary dressing. Disadvantages of hydrogel in contact lenses are lens deposition, hypoxia, dehydration and red eye reactions.
CONCLUSION
The crosslinked polymer networks containing hydrogels that soak up substantial amounts of aqueous solutions. There are large number of novel hydrogel systems have been observed. There are an adequate amount of scientific evidences for the potentiality of hydrogels in delivery of drug molecules to a preferred site by triggering the release through an external stimulus like temperature, pH, glucose or light. These all hydrogels being biocompatible and biodegradable in nature have been used in the progress of nano biotechnology products and have wonderful applications in the field of controlled drug delivery as well.
There have been new inventive methods of hydrophilic polymers preparation and hydrogels that may be employed in the future drug delivery applications. Production of new polymers and crosslinkers with large biocompatibility and better biodegradability would be necessary for victorious applications. In this review, a challenge was prepared, from the materials science point of view, to present an overview of the different classes of hydrogels, hydrogel crosslinking and their characterization. Chemical crosslinking is a highly adaptable method to recover the mechanical property of the hydrogels.
But the crosslinking agents are frequently toxic compounds and not environmental responsive. Physically cross-linked hydrogels are of vast attention for the labile bioactive substances and living cells encapsulation and entrapment, principally when hydrogel development executes in the absence of organic solvents and under mild conditions. To give an approach to the formation of hydrogel systems having a specific command on their microstructure and consequently characteristics thereof, protein engineering may also be valuable.
REFERENCES
1. Okay O. General Properties of Hydrogels, Hydrogel Sensors and Actuators. Springer Series on Chemical Sensors and Biosensors. 2009; 6: 1-14.
2. Hoffman AS. Hydrogels for biomedical applications. Ann N Y Acad Sci. 2001; 944: 62-73.
3. Beneke CE, Viljoen AM, Hamman JH. Polymeric Plant-derived Excipients in Drug Delivery. Molecules. 2009; 14: 2602-2620.
4. In: Markey ML, Bowman ML, Bergamini MY, eds. Chitin and chitosan. London: Elsevier Appl. Sci; 1989:p.713.
5. El-Sherbiny and Yacoub. Hydrogel scaffolds for tissue engineering: Progress and challenges. Global Cardiology Science and Practice. 2013; 3: 38.
6. Katti DS, Lakshmi S, Langer R, Laurencin CT. Toxicity, biodegradation and elimination of polyanhydrides. Advanced Drug Delivery Review. 2002; 54: 933-61.
7. Deo N, Ruetsch S, Ramaprasad K, Kamath Y. Stable environmently sensitive cationic hydrogels for controlled delivery applications. Journal of cosmetic science. 2010; 61: 421-437.
8. Baker JP, Blanch HW, Prausnitz JM. Swelling properties of acrylamide-based ampholytic hydrogels: Comparison of experiment with theory. Polymer. 1995; 36: 1061-1069.
9. Gupta NV and Shivakumar HG. Investigation of Swelling Behavior and Mechanical Properties of a pH-Sensitive Superporous Hydrogel Composite. Iranian Journal of Pharmaceutical Research. 2012; 11(2): 481-493.
10. Pal K, Banthia AK, Majumdar DK. Preparation and characterization of polyvinyl alcohol- gelatin hydrogel membranes for biomedical applications. AAPS PharmSci Tech. 2007; 8: 142-146.
11. Grassi M, Sandolo C, Perin D, Coviello T, Lapasin R, Grassi G. Structural characterization of calcium alginate matrices by means of mechanical and release tests. Molecules. 2009; 14: 3003-3017.
12. Danielssona C, Ruaulta S, Simonetb M, Neuenschwanderb P, Freya P. Polyesterurethane foam scaffold for smooth muscle cell tissue engineering, Biomaterials. 2006; 27: 1410-1415.
13. Mathew F, Soman A, Alias M, Nair SS, joseph J, Vineetha VC, Varghese N, Poosan GV. Hydrogel - A drug delivery device. International Journal of Universal Pharmacy and Bio Sciences. 2014; 3(2): 227-247.
14. El-Sherbiny IM, Abdel-Bary EM, Harding DRK. Preparation and swelling study of a pH-dependent interpolymeric hydrogel based on chitosan for controlled drug release. International Journal of Polymeric Materials. 2006; 55: 789-802.
15. Hong Y, Mao Z, Wang H, Gao C, Shen J. Covalently crosslinked chitosan hydrogel formed at neutral pH and body Temperature. Journal of Biomedical Materials Research. 2006; 79A: 913-922.
16. Kunzier JF. Hydrogels. In: Hoboken NJ, ed. Encyclopedia of polymer science and technology. vol 2. John Wiley & Sons, Hoboken, New Jersey; 2003:p.691.
17. Hennink WE, Nostrum CF. Novel crosslinking methods to design hydrogels. Advanced Drug Delivery Review. 2002; 54(1): 13-36.
18. Sperinde JJ, Griffith LG. Control and predication of gelation kinetics in enzymatically cross-linked poly(ethylene glycol) hydrogels. Macromolecules. 2000; 33: 5467-5480.
19. Chenite A, Chaput C, Wang D, Combes C, Buschmann MD, Hoemann CD, Leroux JC, Atkinson BL, Binette F, Selmani A. Novel injectable neutral solutions of chitosan form biodegradable gels in situ. Biomaterials. 2000; 21: 2155-2161.
NOW YOU CAN ALSO PUBLISH YOUR ARTICLE ONLINE.
SUBMIT YOUR ARTICLE/PROJECT AT editor-in-chief@pharmatutor.org
Subscribe to Pharmatutor Alerts by Email
FIND OUT MORE ARTICLES AT OUR DATABASE